Sugar has been in the headlines of popular media sites, the tagline of diet books, and the sole focus of many blogs over the last decade.
Despite recent interest from mainstream media and the health industry, sugar has been in the spotlight of nutrition research for the last 60 years, and in that time, we have learned a lot about how sugar affects the human body.
This article separates fact from fiction, evidence from hyperbole, and gives you the most informed answer on sugar’s true place in our lives.
READ MORE: about sugar intake, other nutrition tips, and wellness techniques, be sure to check out the NASM nutrition specialist program and see the scope of the course.
What is Sugar?
Before we dive into the health effects of sugar, we first need to understand exactly what sugar is. If we want to get really nerdy, sugar has a very technical definition: “any of the class of soluble, crystalline, typically sweet-tasting carbohydrates found in living tissues and exemplified by glucose and sucrose.”
That definition categorizes many different types of carbohydrates and doesn’t really clarify what most people mean when they talk about sugar. Thus, we are going to simplify it by explaining sucrose, which is the name for common table sugar.
Sucrose is a sugar that comprises of two molecules, glucose and fructose (Figure 1). The different types of sugar we find in our foods are mostly composed of these two molecules, just in different ratios.
For example, table sugar (i.e. sucrose) is 50/50 glucose and fructose, whereas high-fructose corn syrup is 45/55 glucose and fructose.
Read also: Is Fructose Actually Bad for You?
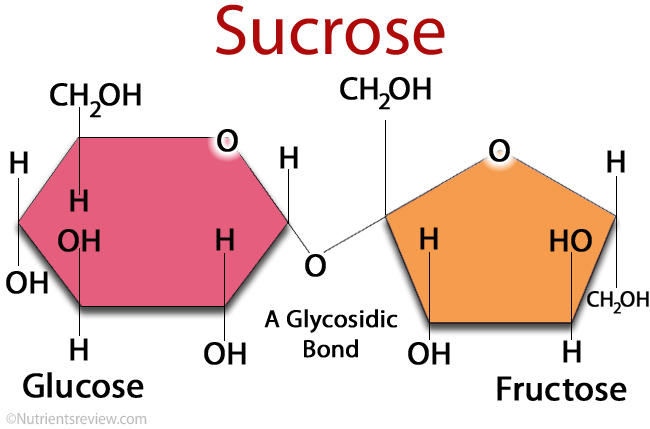
What are the health effects of sugar?
To simplify things, we’ll break this question down into two categories:
- How does sugar contribute to weight gain and why does that matter?
- Is sugar directly harmful to our health?
Is sugar fattening?
There has been a lot of discussion and debate about whether or not sugar contributes to weight gain, and after many decades of research, the truth appears to be that sugar may contribute to weight gain, yet there is no magical property of sugar that makes it a “fattening” food per se.
Sugar increases calorie intake
One of the things we know for certain about weight gain is that calorie surpluses (consuming more calories than you burn) are the key dictators of weight gain.
Average calorie intake increased in the United States between 1971-2006 by roughly 300 calories per day, which mostly comes from added sugars and added fats.
This suggests that sugar may indeed play a role in weight gain as it increases total calorie intake.
Due to this increase of sugar to make foods more pleasant, people are going to a) consume a food that now has a higher calorie content, and b) may be more likely to consume more of that food since it tastes a little better.
This inherent increase of calories can often lead to fat gain if not consumed in moderation.
When people consume food containing added sugar, they tend to consume more calories. However, when people consume sugar just by itself, they do not consume large amounts of it.
A study completed by Richter and Campbell of Johns Hopkins University and published in the Journal of Nutrition in 1940 found that when given access to pure raw sugar separate from their food, rodents consumed the same amount of calories as they did when there was no sugar present. They found that it is the combination of sugar mixed in with the food that makes them consume more calories.
Ok, so we know that adding sugar to food can increase our calorie consumption which can lead to weight gain. What about the direct fattening effects of sugar?
Sugar, by itself, is not fattening
It turns out that sugar by itself is not inherently fattening, and many studies have shown this to be true.
For example, in one report, people were fed high sugar diets (43% of their total daily calories were from sugar), but were held in a calorie deficit. These people lost weight over the span of 6 weeks.
In another study, people were given a high sugar liquid drink as their diet and lost substantial amounts of weight (one participant lost over 200 pounds) as the participants consumed fewer calories due to the fact the liquid drink was not very tasty.
To wrap this section up, here is what we know about sugar and weight gain: when we add sugar to our food, we often increase our total calorie intake which can lead to weight gain; however, sugar alone is not an essentially fattening thing to consume. If you consume the same amount of calories with different amounts of sugar in your diet, there will be no real difference in weight gain or weight loss.
Why does weight gain matter for health?
Why do we care about how sugar might impact weight gain? Well, it turns out that weight gain, and weight loss, make a huge impact on your health. The risk of diabetes, heart disease, and many forms of cancer increase as your body weight and body fat increase.
For example, your lifetime diabetes risk increases from 7.6% for underweight people to 70.3% for very obese people. Additionally, the lifetime risk of having a cardiovascular event is 21% and 67% higher for overweight and obese people, respectively, compared to people at a healthy weight.
This means that weight gain is a great contributor to many dangerous health risks. Sugar cannot be fully blamed for weight gain, which primarily results from a calorie surplus over long periods of time. However, sugar intake can be one piece of a larger lifestyle that consists of an excessive daily calorie intake.
Does sugar directly hurt our health?
We just showed that sugar can be a contributing factor to caloric surpluses, leading to weight gain, but sugar alone is not considered a fattening nutrient. Further, we showed that weight gain leads to potentially dangerous health outcomes (Figure 2).
Now, another important question to answer is whether or not sugar has a directly harmful effect on our health.
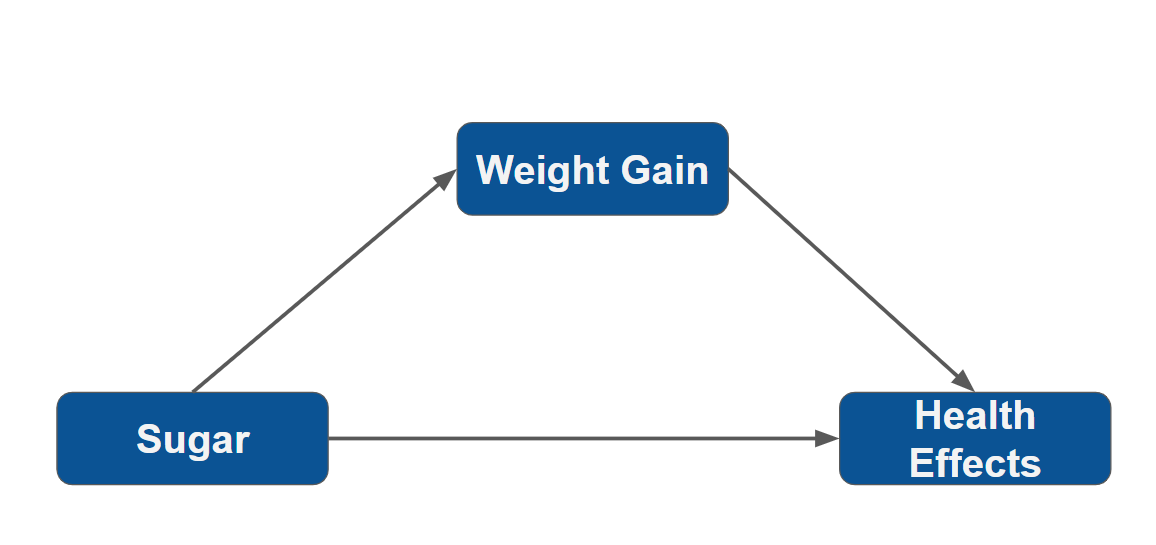
The answer is… drumroll please… maybe.
Here is what we currently know about sugar and its effects on the body.
High sugar intake is widely known as being associated with heart disease, cancer, and diabetes. However, this information needs to be interpreted with a bit of caution.
People who consume large amounts of sugar also tend to have a cluster of other unhealthy behaviors that are associated with heart disease, such as: drinking, smoking, being overweight, low physical activity, and high blood pressure.
More importantly, many of the associations with sugar only appear once the sugar levels are above a certain threshold, often in amounts that have exceeded a reasonable moderate intake.
When we look past the observational data discussed above, actual randomized trials of sugar intake and health outcomes do not appear as clear.
For example, in heart disease, most of these studies show no real effect of moderate-to-above-average sugar intake when the sugar intake is consumed in the context of a normal calorie intake (meaning people are not consuming large numbers of calories).
Researchers may struggle to draw conclusions regarding sugar’s impact on disease because it is often difficult to conduct research on humans and their sugar intake. To properly study and confirm all of its effects would require a vast number of people, several decades of highly controlled experimentation, and fairly invasive procedures.
Due to this fact, we rely pretty heavily on the observational data we have mentioned above and a handful of smaller, shorter, clinical trials.
Much of the “deep science” we know about sugar and heart disease comes from experiments we have done on rodents and non-human primates (i.e. mice and monkeys). However, these studies may not be very informative as mice and monkeys succumb to diseases and process sugar differently than humans do.
While these studies have helped us develop some helpful therapies for heart disease, they don’t tell us much about the human disease and we can’t really say much about whether sugar is bad for you based on that type of evidence.
Most of the evidence we have from humans suggest that sugar, per se, does not exert measurable consequences on our health when consumed in modest amounts in the context of an otherwise healthy lifestyle. Furthermore, most evidence tends to suggest that moderating intake of sugar is advisable as there is little downside and may provide a minor health benefit.
TL;DR
We can take decades of research and thousands of books and articles and distill them down to a few simple truths:
- Sugar does not appear to be inherently fattening compared to other forms of carbohydrates.
- Sugar can contribute to weight gain when added sugars contribute to increased calorie intake.
- Sugar does not appear to have any substantial direct negative health effects.
- Much of the negative health effects with regard to heart disease, diabetes, and cancer appear to be due to weight gain that is often associated with high calorie, fat, sugar diets.
- Moderating sugar intake is pertinent advice as reducing sugar intake to below the average may provide some modicum of benefit on one’s health and there are no negative side effects from doing so.
- Sugar does not need to be demonized as a nutrient. It can be consumed in modest quantities with no real negative effects.
Has this blog piqued your interest in nutrition? Consider becoming a Certified Nutrition Coach, you can learn more by clicking here.